Exploring 12V to 100V Boost Converters: Principles and Applications
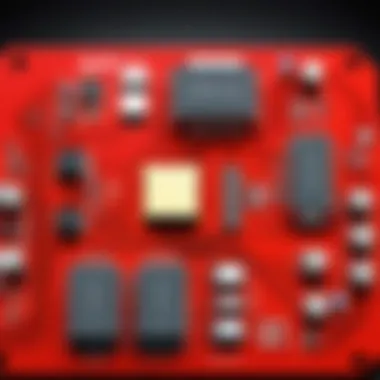
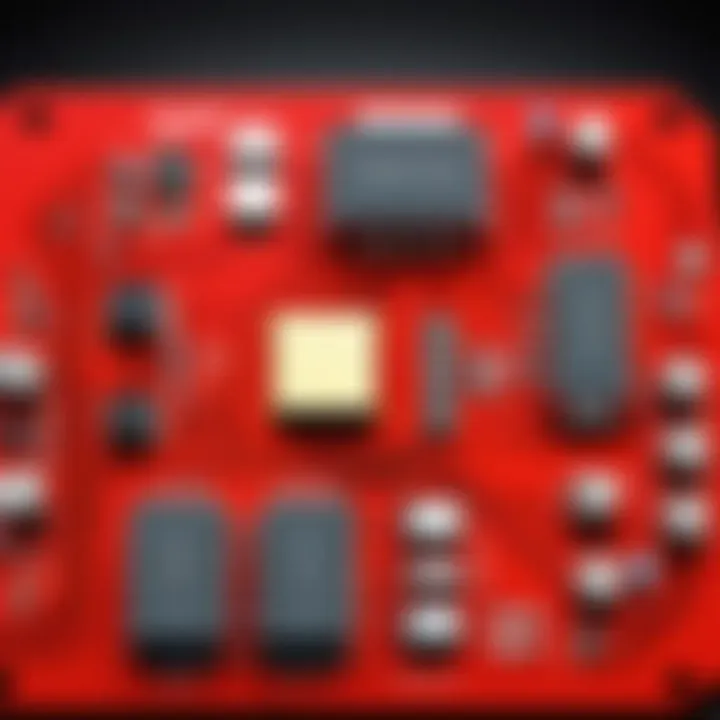
Intro
Boost converters play an essential role in modern electronics, enabling engineers and tech enthusiasts to achieve higher voltage levels from a lower input voltage. Specifically, the 12V to 100V boost converter has significant importance across various applications such as renewable energy systems, automotive electronics, and consumer devices. Understanding the operational principles, design considerations, and market trends related to these devices is vital for anyone looking to explore or implement this technology.
The landscape of power electronics is rapidly evolving, with increasing demands for energy efficiency and compact designs. In this article, we aim to provide a comprehensive overview that caters to both novices and experienced users. By delving into the workings of boost converters, we will highlight their relevance in today’s technological advancements.
This exploration encompasses product features, installation procedures, performance evaluations, and potential market implications. Our goal is to equip readers with the knowledge to navigate the complexities of boost converters, while also facilitating informed decisions when considering these components for specific applications.
Product Overview
Boost converters function by converting a lower DC voltage to a higher DC voltage. They accomplish this using various electronic components, including inductors, switches, and diodes. The design of a boost converter greatly influences its efficiency and effectiveness in different scenarios.
Key Features
- High Efficiency: Modern boost converters can achieve efficiency rates often exceeding 90%, ensuring minimal energy loss.
- Compact Design: Many models are designed for space-saving installations, making them ideal for applications with limited physical space.
- Wide Input Range: These converters can accept a range of input voltages, allowing flexibility in various setups.
Technical Specifications
Key specifications vary among products but typically include:
- Input Voltage Range: 12V to 100V
- Output Voltage: Adjustable or fixed outputs, often specified in model data sheets.
- Current Rating: Defines the maximum output power.
- Switching Frequency: Impacts the size of passive components and overall efficiency.
Pricing Information
The cost of 12V to 100V boost converters varies widely based on specifications and features. Basic models can start as low as $10, while advanced versions with extensive capabilities can exceed $100. Evaluating the required features will help determine a suitable investment based on the intended application.
Performance Analysis
Understanding how these converters perform under various conditions is critical in choosing the right option.
Benchmark Results
Performance benchmarks often compare efficiency, thermal management, and load regulation capabilities. For instance, under standard testing conditions, some models demonstrate an efficiency of 95%, indicating high performance under load.
Real-World Use Cases
- Renewable Energy Systems: When integrating solar panels, these converters maximize the energy harvested from varying voltage levels.
- Electric Vehicles: Boost converters are used to manage battery voltages for driving motors efficiently.
- Consumer Electronics: Products like power banks often utilize these converters to provide higher output for faster charging.
Comparison with Competitors
While examining boost converters, it is essential to compare them with other similar products in the market. Notably, manufacturers such as Texas Instruments and Analog Devices provide extensive product lines with varying efficiencies and features. Identifying a superior option often involves evaluating performance data and cost-effectiveness.
Installation and Setup
Installing a boost converter requires careful planning. A systematic approach can mitigate common issues.
Required Tools and Components
- Soldering Iron
- Multimeter
- Input and output capacitors
- Inductor (aligned with the specifications of the converter)
Step-by-Step Installation Guide
- Gather necessary tools and components.
- Ensure the workspace is free of potential hazards.
- Connect the input voltage source to the converter, following polarity.
- Attach the output components ensuring correct orientation.
- Power on the system and monitor output voltage.
Troubleshooting Common Issues
- If the output voltage is unstable, check input connections and ensure no loose connections exist.
- Excessive heating can indicate inefficiencies; verify component ratings align with expected load.
Pros and Cons
Boost converters come with their advantages and drawbacks that must be considered.
Advantages of the Product
- Compact and efficient voltage conversion.
- Versatility across multiple applications.
- Ability to operate under varying load conditions.
Disadvantages or Limitations
- Potential heat generation requiring effective thermal management.
- Efficiency may decrease under heavy loads or higher output requirements.
Who It’s Best Suited For
These converters are ideal for engineers designing portable electronics, renewable energy systems, and automotive applications needing efficient voltage regulation.
Final Thoughts
In summary, 12V to 100V boost converters are vital in optimizing electrical systems across numerous sectors. Understanding their key features, performance metrics, and installation practices can significantly enhance their implementation.
Recommendations for Potential Buyers
Assess specific needs in terms of voltage and current requirements before purchasing. Focus on models with proven performance benchmarks and user-friendly designs.
Future of the Technology or Product Line
As technology progresses, innovations in boost converter designs may emerge. Improved efficiencies and reduced sizes are trends that will shape future offerings. Continuous advancements in electronics signify that these converters will become even more integral to energy management systems.
Foreword to Boost Converters
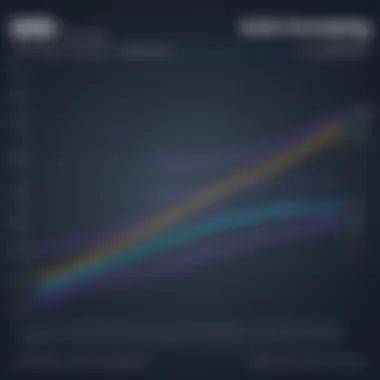
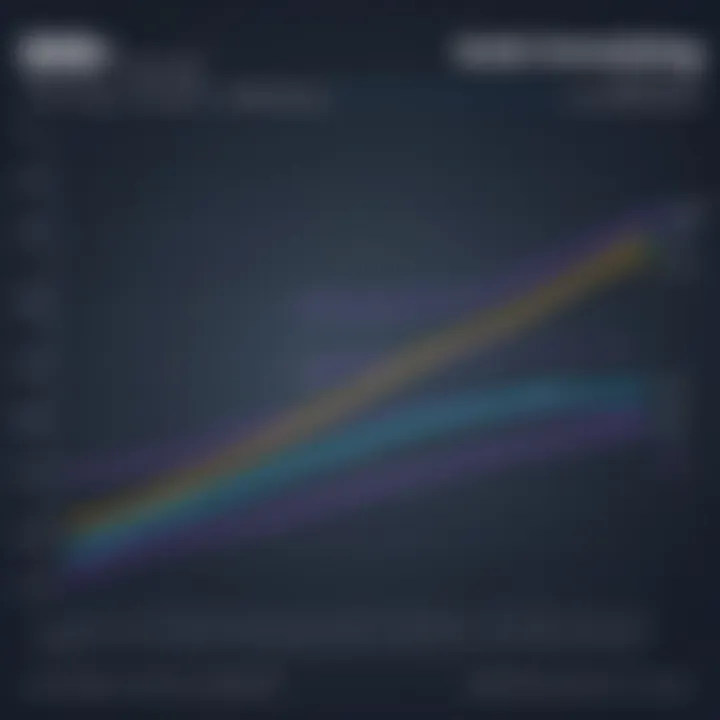
Boost converters play a crucial role in modern electrical and electronic systems. They allow for the efficient stepping up of voltage from a lower level to a higher level. This fundamental capability is important because many devices and applications require specific voltage levels to function optimally. The boost converter is an essential part of power management in various industries such as renewable energy, consumer electronics, and electric vehicles.
Definition and Purpose
A boost converter is a DC-DC converter that increases an input voltage to a higher output voltage. It works by storing energy in an inductor and releasing it at a higher voltage. The primary purpose is to provide a stable output voltage while drawing power from a lower voltage source. This functionality is particularly prominent when dealing with batteries, where the voltage must be increased for certain applications.
Key benefits of boost converters include:
- Efficiency: They can achieve high efficiency rates, minimizing power loss.
- Versatility: They can work with various input voltages, adapting to different power supplies.
- Compact Design: Most boost converters are designed to take up minimal space, making them suitable for portable devices.
Understanding boost converters enables engineers and enthusiasts to design efficient power systems that meet specific energy requirements.
How Boost Converters Work
The operation of boost converters revolves around its key components: inductor, switch, diode, and output capacitor. The main process can be broken down into two phases: charging the inductor and discharging it.
- Charging Phase: The switch is closed, causing current to flow through the inductor. This current flow stores energy in the magnetic field of the inductor, and the voltage across the inductor rises.
- Discharging Phase: When the switch opens, the inductor resists the sudden drop in current. It releases the stored energy through the diode to the output capacitor and load. The output voltage increases because the inductor's voltage adds to the input voltage.
This sequence continues, regulated by a control system that manages the duty cycle of the switch. The more time the switch remains closed, the more energy is transferred. However, it is essential to maintain balance to avoid overloading or overheating.
Boost converters are essential for improving the efficiency of power supplies in diverse applications, highlighting their importance in contemporary technology.
In summary, the functioning of boost converters is a synergy of energy storage and conversion processes. Being aware of how these components work together is vital for optimizing electrical systems.
The Importance of Voltage Conversion
Voltage conversion is a critical topic in the field of electrical engineering and technology. The capability to adjust voltage levels allows devices to operate efficiently, addressing diverse power requirements across various applications. Understanding the necessity of this conversion helps in grasping how systems like boost converters function effectively in real-world scenarios.
When we consider voltage conversion, we recognize its fundamental role in optimizing performance and ensuring the safety of electrical systems. Devices designed to manage different voltage levels play a vital part in enhancing energy efficiency. This aspect is particularly highlighted with boost converters that increase voltage from 12V to 100V. Their relevance becomes significant in contexts where high voltage is crucial for operation, such as in renewable energy systems and advanced consumer electronics.
Furthermore, the capability of voltage conversion impacts the overall reliability and longevity of electronic systems. With properly managed voltage, the risk of damage to sensitive components is greatly mitigated. Designers focus on selecting the appropriate converters based on specific voltage requirements to maintain optimal operation. Thus, recognizing the importance of voltage conversion is essential not only for technical applications but also for the broader efficiency and sustainability goals in modern technology.
Applications of Voltage Conversion
Voltage conversion finds its applications in various industries, impacting both common consumer devices and industrial equipment. Below are some primary areas where these conversions are crucial:
- Power Supply for Renewable Energy: Solar panels often produce 12V, while inverters may require higher voltages to feed energy into the grid. Boost converters enable this transition effectively.
- Consumer Electronics: Many devices, like LED lighting and laptops, require different voltage inputs. Boost converters allow for compact design where the required voltage can be achieved without size constraints on components.
- Electric Vehicles: The battery systems in electric vehicles typically utilize lower voltages but need to interact with high-voltage systems for propulsion and energy management.
- Telecommunications: Modern communication devices require consistent voltage levels to transmit data effectively, especially over long distances where loss can occur.
The diverse applications underscore the necessity of voltage conversion, demonstrating its significance across various sectors.
Why 12V to 100V is a Key Focus
The 12V to 100V range is particularly notable because it addresses many common challenges in today's technological landscape. Understanding why this range is a focal point reveals insights into practical applications and advantages:
- Broad Compatibility: Many devices and systems operate efficiently within the 12V range. Boosting up to 100V makes these systems versatile and interoperable with higher-voltage applications.
- Energy Efficiency: Higher voltage levels generally contribute to reduced current, thus minimizing energy loss due to resistance in wires. This enhances performance in energy-intensive applications.
- Design Flexibility: Engineers have more leeway when designing circuits. A 12V input can be adjusted to meet the demands of various components, achieving desired performance without requiring frequent redesigns.
- Emphasis on Sustainability: As technology advances, there is a push towards renewable sources. The capability to efficiently convert and manage voltage helps integrate renewable energy systems into existing infrastructures seamlessly.
Overall, focusing on the 12V to 100V range highlights how essential voltage conversion is to modern engineering and design considerations. This balance between practical application and technological advancement holds considerable potential for future developments.
Technical Specifications
Understanding the technical specifications of boost converters is crucial for selecting the right device for specific applications. This section covers essential elements such as voltage ranges and efficiency, which are key factors that influence performance and usability in real-world scenarios. Knowing these specifications helps engineers and enthusiasts make informed choices, optimize system designs, and troubleshoot issues effectively.
Input and Output Voltage Ranges
When working with boost converters, the input and output voltage ranges determine their suitability for various applications. For example, a converter designed to step up from 12V to 100V must handle those specific input and output levels reliably.
Input Voltage Range
The input voltage range is the minimum and maximum voltage that can be applied to the converter. This range must be compatible with the power source to ensure proper functionality. Typically, a 12V boost converter can operate efficiently within a narrow voltage span, often around 7V to 15V. However, devices may also support a wider range, allowing flexibility in their applications.
Output Voltage Range
The output voltage is equally important. The converter must produce a stable output voltage, especially when it is expected to deliver 100V in this case. It is essential that the output voltage matches the requirements of the load connected to the converter, whether it is for consumer electronics or other systems.
An optimal voltage range ensures efficiency and reliability in various applications.
To construct a useful voltage range, factors such as load conditions, energy consumption, and thermal limits must also be considered. These conditions can affect both efficiency and performance. In some instances, bursts of high demand can require a higher output voltage, which must be addressed in the converter's design.
Efficiency and Performance Metrics
Efficiency and performance are critical metrics that reflect a boost converter’s capability to convert power with minimal loss. These measurements impact not only the effectiveness of the device but also its viability for different projects. Design engineers often prioritize a high-efficiency rating to maximize system performance.
Measuring Efficiency
Efficiency is calculated as the ratio of output power to input power. A higher efficiency means less energy is wasted as heat, which is vital in sensitive applications. For instance, boost converters often achieve efficiencies ranging from 80% to over 95% under optimal specified conditions. Variability in this metric can arise from load and input fluctuations, as well as component selection.
Performance in Real Scenarios
Real-world performance metrics include switching frequency, transient response, and thermal performance. Switching frequency, for example, can affect the size of passive components used in the circuit. A high frequency may allow for smaller inductors and capacitors but could introduce switching losses. The transient response showcases how quickly a converter can adjust to changes in load or input conditions, directly influencing its reliability in dynamic environments.
Moreover, thermal performance is critical since excessive heat can degrade components, leading to efficiency loss. Manufacturers often recommend heat sinks or specialized layouts to mitigate these issues. Thus, understanding these specifications is paramount to leveraging boost converters effectively across various applications.
Common Types of Boost Converters
Boost converters are vital components in modern electronic systems. Two primary types of boost converters can optimize power management and energy efficiency: Step-Up Converters and Buck-Boost Converters. Each type plays a crucial role in specific applications and possesses unique characteristics.
Step-Up Converters
Step-up converters, also known as boost converters, are designed to increase voltage levels from a lower voltage source, such as 12V, to a higher output, up to 100V or beyond. These converters are essential in various applications where voltage needs to be raised for effective operation.
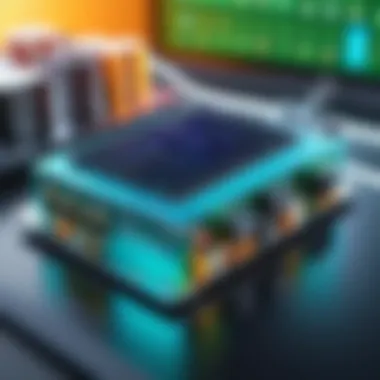
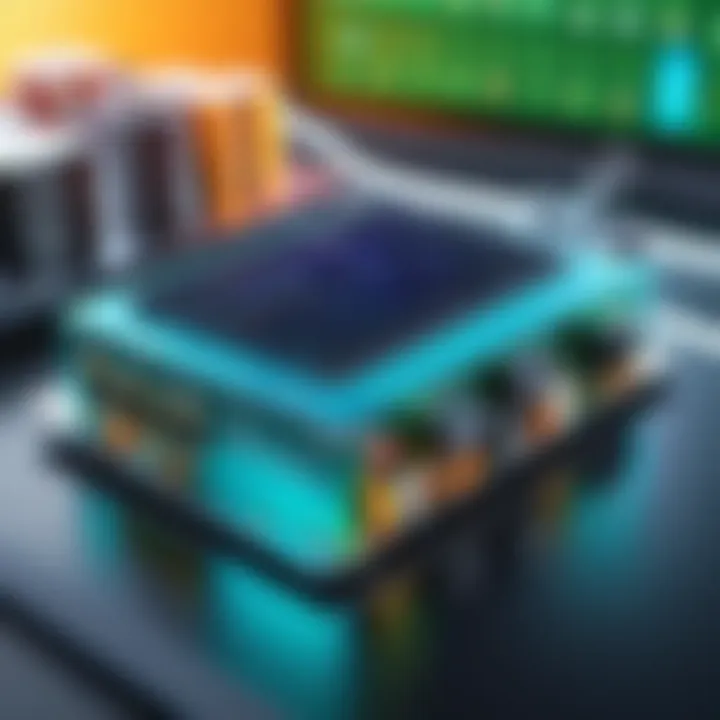
They utilize an inductor, a switch, a diode, and a capacitor in their design. The basic operation involves storing energy in the inductor when the switch is closed and releasing that energy to the output when the switch opens. This simple yet effective mechanism allows for smooth voltage scaling while maintaining good efficiency.
One notable advantage of step-up converters is their ability to power devices that require higher voltages without needing larger power supplies. For instance, in renewable energy systems, these converters can efficiently boost the energy harvested from solar panels to levels suitable for conventional electrical grids.
However, they also have considerations, such as an increase in current drawn from the input. Thus, careful attention to current capability and thermal management is necessary for optimal performance.
Buck-Boost Converters
Buck-boost converters are a unique type of voltage converter that can both increase and decrease voltage, providing flexibility in applications requiring varied voltage levels. This versatility is especially beneficial when the input voltage can fluctuate around the desired output level.
The operation of buck-boost converters can be slightly more complex than step-up converters. They can work in two primary modes: buck (step down) and boost (step up). By using a combination of inductors, capacitors, and switches, they adjust the voltage as needed based on system demands.
A key benefit of buck-boost converters is their adaptation to diverse energy sources. For example, in battery systems, where voltage can change, these converters allow devices to function optimally regardless of the battery state. They are widely used in portable electronic devices, electric vehicles, and power management solutions.
However, they are often less efficient than step-up converters in certain scenarios, which may limit their application in highly efficient systems. Careful design is necessary to optimize their performance.
Circuit Design and Components
The design of a boost converter circuit is critical for its efficiency and functionality. Understanding circuit design is essential for optimizing performance and reliability in various applications. In this section, we will discuss the important components that make up a boost converter and how their arrangement affects the overall operation.
Essential Components of Boost Converters
A boost converter is composed of several key components that work together to increase voltage levels. These components include the inductor, switch, diode, capacitor, and controller.
- Inductor: The inductor stores energy when the switch is closed and releases it when the switch opens. Inductor selection is crucial, influencing efficiency and size. A poorly chosen inductor can lead to losses, making proper sizing essential.
- Switch: Typically implemented using transistors such as MOSFETs, the switch controls the energy transfer by toggling between open and closed states. The switching speed impacts efficiency; faster switching can yield better performance but may also generate more heat.
- Diode: The diode allows current to flow in one direction, helping to prevent back current when the switch is off. Schottky diodes are preferred in many applications due to their faster recovery time and lower forward voltage drop.
- Capacitor: Capacitors smooth out the output voltage and store charge. It is essential to select a capacitor that can handle the ripple voltage and provide stable output.
- Controller: The controller manages the switching of the device based on feedback from the output. Advanced control methods, such as peak current control and voltage mode control, enhance the performance of the converter.
Each component's voltage ratings, current capabilities, and thermal characteristics must align with the overall requirements of the application. The interrelation of these components is essential; a failure in one can lead to the inefficiency or malfunctioning of the entire system.
Schematic Design and Layout
The schematic design is a visual representation of the circuit. It illustrates how components connect and interact in the boost converter, providing insight into its operation. A good schematic serves as a blueprint for building the circuit and helps in diagnosing issues.
When designing a schematic, consider the following:
- Component Placement: Components should be placed thoughtfully to minimize parasitic inductance and capacitance. This can improve overall circuit performance and reduce noise.
- Feedback Loop: A well-designed feedback loop is vital for maintaining voltage regulation. Properly placing components related to the feedback can ensure a quick response to changes in load or input voltage.
- Grounding: Grounding techniques can significantly influence performance. A solid ground path reduces noise and provides stability.
- Thermal Management: When laying out the design, account for the heat generated by components, especially the switch and diode. Place heat sinks in areas where airflow is maximized.
A neat and organized schematic facilitates troubleshooting and adjustments during the testing phase. It is also essential for future modifications or upgrades.
Conclusion: Proper circuit design and component selection are fundamental for the effective operation of boost converters. Careful attention to detail in schematic design can lead to enhanced performance and reliability. Understanding these elements is crucial for anyone involved in working with 12V to 100V boost converters.
Control Methods in Boost Converters
In the realm of boost converters, control methods are essential for ensuring proper functionality and performance. These methods determine how the converter responds to changes in input voltage, load conditions, and output requirements. Understanding these control strategies is crucial for both designers and users, as they influence efficiency, stability, and overall performance.
Voltage Mode Control
Voltage Mode Control (VMC) is a prevalent control method in boost converters. This technique regulates the output voltage by adjusting the duty cycle of the switch. The basic idea is simple: if the output voltage drops below a set reference, the controller increases the duty cycle to push more energy into the output. Conversely, if the output rises above the desired level, the duty cycle decreases, maintaining a stable output.
One key advantage of Voltage Mode Control is its simplicity, leading to cost-effective designs. However, it must be noted that VMC can face stability issues under rapidly changing load conditions.
- Benefits of Voltage Mode Control include:
- Straightforward implementation
- Lower component count reduces costs
- Suitable for specific applications with stable load requirements
Despite its advantages, Voltage Mode Control can be less responsive to fast transients. Users must consider this limitation when designing systems that experience variable loads.
Current Mode Control
In contrast, Current Mode Control (CMC) offers a more dynamic approach to managing boost converter operation. CMC monitors both the output voltage and the inductor current. This dual feedback loop allows for quick adjustments to the switch duty cycle based on changes in load or input conditions.
With Current Mode Control, the converter is capable of reacting faster to transients, leading to enhanced performance in fluctuating environments.
- Benefits of Current Mode Control include:
- Improved transient response
- Enhanced stability under varying load conditions
- Built-in current limiting protection
This method also has its drawbacks. Current Mode Control can be more complex to implement and requires careful compensation to ensure stability. Additionally, it may necessitate more components, potentially increasing the overall size and cost of the converter.
Both control methods aim for high efficiency in boost converter designs but cater to different application needs. Understanding each method's strengths and weaknesses is vital for optimizing performance.
In summary, Control Methods in Boost Converters play a decisive role in ensuring effective voltage regulation and system stability. Vigilant selection between Voltage Mode Control and Current Mode Control depends on the specific requirements of the application, balancing cost, performance, and complexity.
Challenges and Limitations
Boost converters play a crucial role in modern electrical engineering by efficiently stepping up voltage levels. Yet, they are not without their challenges and limitations. Understanding these drawbacks is essential for both manufacturers and users alike to ensure optimal performance in various applications.
Heat Dissipation Issues
Heat management is a significant concern for boost converters. As these devices convert lower voltage to higher voltage, they generate heat during the process. This heat, if not properly managed, can lead to reduced efficiency and even potential device failure.
Key aspects to consider include:
- Component Selection: Choosing components with better thermal performance can mitigate heat generation.
- Heat Sinking: Effective heat sinks can dissipate heat, maintaining optimal operating temperatures.
- Thermal Design: A well-planned circuit layout helps in heat distribution and management.
Addressing heat dissipation not only improves the longevity of the device but also ensures sustained operational efficiency. Thus, paying attention to thermal management is a necessity rather than an option.
Ripple Voltage Concerns
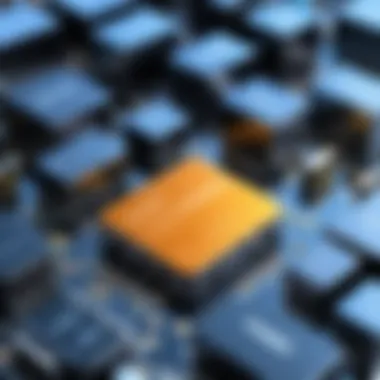
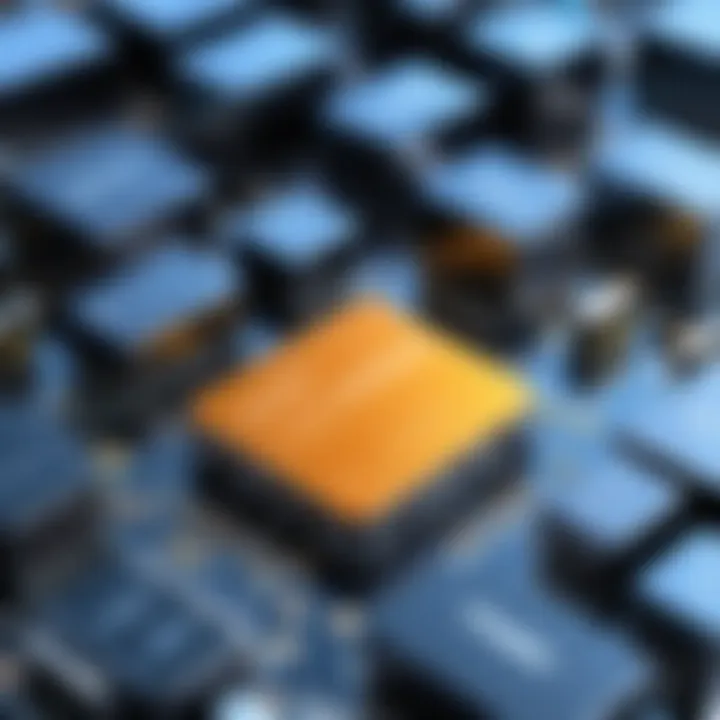
Ripple voltage is another challenge encountered in boost converters. It refers to the small, unwanted fluctuations in output voltage. This voltage variation can affect performance in sensitive applications, leading to noise issues and potential failure in electronic circuits.
Considerations regarding ripple voltage include:
- Capacitor Quality: Higher quality output capacitors can effectively reduce ripple.
- Inductor Design: Choosing the right inductor can help maintain constant output.
- Feedback Control Systems: Implementing sophisticated feedback mechanisms can stabilize output voltage.
Managing ripple voltage is essential for enhancing the reliability of boost converters. Users must carefully select components and design circuits that account for these fluctuations to achieve more stable performance in various applications.
Understanding the challenges and limitations of boost converters is vital for optimizing their performance in practical applications.
Boost Converter Applications
Boost converters are pivotal in various industries, enabling efficient voltage elevation for numerous applications. Understanding their applications is essential for grasping their significance in technology and society. The growing demand for efficient power conversion methods is a driving force behind innovation, making knowledge of these systems invaluable for tech enthusiasts and engineers alike.
Renewable Energy Systems
Renewable energy systems rely heavily on boost converters to efficiently convert low DC voltages to higher levels needed for effective power distribution and storage. Solar panels, for instance, often produce voltage in the range of 12V. However, to send this power into the grid or to charge batteries, the voltage must be stepped up. Boost converters can achieve this while maintaining a high efficiency, ensuring minimal energy loss.
Furthermore, this technology is crucial in wind energy systems where the output voltage fluctuates. A boost converter helps stabilize the voltage, enabling consistent performance and integration into the grid. The versatility of boost converters makes them suitable for various renewable sources, enhancing the overall efficiency of these systems.
Consumer Electronics
In the realm of consumer electronics, boost converters are essential for devices that require higher voltages to function effectively. Many portable devices, such as smartphones and tablets, depend on these converters to maximize battery life. When the battery voltage drops, a boost converter steps it up, allowing the device to operate continuously without significant performance loss.
Additionally, LED lighting systems often utilize boost converters to achieve the desired brightness levels. They allow the use of lower voltage sources while ensuring the LEDs operate efficiently at higher voltages. This application not only provides better luminosity but also extends the lifespan of the lighting systems by managing voltage levels effectively.
Electric Vehicles
Electric vehicles (EVs) are at the forefront of the movement toward sustainable transportation. Boost converters integrate into EV designs, particularly for managing power from the battery pack to the electric motor. The battery typically operates at a lower voltage, but the motor often requires a higher voltage for optimal performance.
Boost converters facilitate this transition, allowing EVs to utilize their batteries more efficiently. With the rising emphasis on longer ranges and faster charging, these converters play a crucial role in the design of battery management systems. They contribute significantly to the overall performance and feasibility of electric cars in the market.
Boost converters enhance the efficiency and functionality of renewable energy systems, consumer electronics, and electric vehicles, driving forward the push for better technologies.
Emerging Trends in Boost Converter Technology
In the rapidly changing landscape of electrical engineering, understanding the emerging trends in boost converter technology is crucial. These trends not only enhance the functionality of devices but also pave the way for innovative applications. By focusing on advancements in efficiency and integration with smart technologies, we can grasp how these trends significantly influence the future of power electronics.
Advancements in Efficiency
Improving the efficiency of boost converters is a primary concern for manufacturers and engineers. High efficiency translates to lower energy losses, leading to reduced operational costs and enhanced performance in various applications.
Recent research showcases a range of advancements that focus on increasing efficiency. These include:
- Use of Improved Switches: Modern materials such as gallium nitride (GaN) are being used to replace traditional silicon. GaN devices offer faster switching speeds and lower conduction losses.
- Enhanced Control Algorithms: New control methods provide better regulation and management of energy flow, allowing converters to operate closer to their optimal efficiency points.
- Thermal Management Techniques: Better heat dissipation strategies reduce thermal stress on components, contributing to longer lifespans and sustained efficiency.
Overall, these advancements not only lead to highly efficient converters but also enable broader application possibilities in sectors like renewable energy and electric vehicles.
Integration with Smart Technologies
Integration with smart technologies represents another significant trend in boost converter development. The rise of Internet of Things (IoT) devices and smart grids necessitates a sophisticated approach to voltage regulation. Boost converters are increasingly designed to facilitate seamless communication and efficient power management in these smart environments.
Some key elements of this integration include:
- Smart Control Systems: Boost converters now incorporate smart control systems that allow for real-time monitoring and adaptive management of power levels. This feature is essential for maintaining system stability.
- Connectivity: Enhanced connectivity solutions enable devices to communicate their status and power needs with other systems, fostering greater efficiency in energy distribution.
- Automated Adjustments: With built-in intelligence, these converters can automatically adjust their output based on dynamic load changes and power conditions.
The integration of boost converters with smart technologies marks a shift towards a more connected and efficient energy ecosystem. This adaptability is critical for meeting the future demands of global energy consumption.
In summary, the trends in boost converter technology highlight the ongoing commitment to efficiency and integration. As these technologies continue to evolve, they will play a key role in shaping the future of various industries that rely on effective voltage conversion.
Market Overview of Boost Converters
Understanding the market for boost converters is critical for recognizing their relevance and influence in various industries. This section delves into several aspects of the market landscape, including key manufacturers and their product offerings, as well as pricing trends that affect both consumers and businesses.
Key Manufacturers and Products
The boost converter market features a diverse range of manufacturers, each providing unique solutions tailored to specific applications. Some of the prominent players in this field include:
- Texas Instruments: Known for a comprehensive portfolio of boost converters that focus on efficiency and reliability.
- Analog Devices: Provides advanced solutions with a special emphasis on high performance in compact designs.
- Maxim Integrated: Offers a variety of power management devices with integrated features that optimize space and performance.
- Infineon Technologies: Focuses on sustainable solutions, aimed at powering the growing market for electric vehicles.
- Microchip Technology: Offers versatile options suitable for consumer electronics and industrial applications.
These manufacturers often position their products as solutions for challenges faced in energy conservation and efficiency. For example, the LM2577 from Texas Instruments is a popular choice among hobbyists due to its straightforward implementation and versatile application.
Pricing Trends
The pricing of boost converters can vary significantly based on several factors such as performance specifications, brand reputation, and overall market demand. Here’s a simplified overview of the pricing dynamics:
- Entry-Level Products: Basic models can range from $1 to $5, suitable for low-power applications and hobbyist projects.
- Mid-Range Options: More advanced products typically cost between $5 to $20. These often offer better efficiency, handling higher currents.
- High-End Solutions: Specialized converters may exceed $20, incorporating advanced features such as thermal management and built-in protections suitable for industrial or renewable energy systems.
The pricing strategy in this sector is heavily influenced by the increasing demand for efficient energy solutions across industries, leading manufacturers to invest in R&D for innovations that command higher prices.
Ending and Future Outlook
Summary of Key Points
In this article, we explored the fundamentals of boost converters, specifically the transition from 12V to 100V systems. We discussed various applications, which range from renewable energy to electric vehicles. Understanding the importance of efficiency and performance metrics is crucial. Boost converters enhance voltage levels to meet device requirements for various technologies. The design aspects and technical specifications of these systems were thoroughly outlined, providing insights into optimal circuit configurations and components necessary for effectiveness.
Key points include:
- Definition and purpose of boost converters.
- Operational principles, including voltage and current mode control.
- Challenges such as heat dissipation and ripple voltage.
- Current market trends and future developments in technology.
Potential for Future Developments
The future of 12V to 100V boost converters holds significant potential. With increasing demand for efficient energy solutions, there is an ongoing push towards enhancing efficiency levels. Innovations in semiconductor technology may pave the way for smaller, more efficient components that can operate at higher voltages without significant loss.
Integration with smart technologies will also be a key area. This can involve IoT devices that optimize energy consumption in real-time. As industries move toward automation, the incorporation of artificial intelligence and machine learning in control systems can lead to smarter boost converters. In summary, the outlook for the sector remains promising with advancements in technology and rising energy demands. Boost converters will undoubtedly play a pivotal role in future energy solutions.